Shining a light on light bosons
Several currently unsolved mysteries in physics might be explained by the existence of as-yet-unknown particles. Of the various kinds of hypothetical particles that are possible, a subset known as “light bosons” are one of the more promising.
Perimeter Faculty member Junwu Huang is on the hunt for these elusive particles.
To find them, he is testing out new low-cost, small-scale experiments, which are meant to serve as a complement to expensive international particle-hunting experiments like the Large Hadron Collider. As part of his efforts, Huang is spearheading a brand-new initiative in small-scale experiment design at Perimeter Institute, bringing together a collection of theoretical and experimental experts to accelerate progress in the field.
“In the last 10 to 15 years, the precision of some small-scale detectors has increased by orders of magnitude – that opens up a lot of new opportunities to search for new particles,” Huang says.
What is a light boson?
Several hypothetical particles, including the dark photon and the axion, fall under the category of light bosons. These particles have an extremely tiny mass (many orders of magnitude smaller than an electron), hence the “light” in their name. These bosons can also be force carriers. Photons, for example, are bosons that carry the electromagnetic force, while gluons are bosons that carry the strong nuclear force, and so on.
A dark photon, if it exists, would transmit an as-yet-undetected “dark electromagnetic force.” But, unlike a regular photon, it would a have mass – albeit a very small one. Similarly, an axion would mediate its own as-yet-undetected force. Axions are of particular interest to physicists because they might have the exact properties needed to solve a long-standing physical problem known as the “strong CP problem.”
What is the Strong CP Problem?
When particles interact, the interaction should conserve some properties held by the original particles. For example, charge conjugation (C) is a property that distinguishes a particle from its antiparticle, while parity (P), is a property describing the mirror image of a particle’s orientation in space. In electromagnetism, these properties are indeed conserved, but they are not always conserved in weak nuclear interactions (i.e., radioactive decay).
In theory, CP symmetry violation should also be possible in the strong nuclear interaction (which binds the nucleus of atoms together), but no experimental evidence for this has been found. Particle physicists wonder if there might be something preventing CP symmetry violation in strong interactions, and that something might be a new particle: the axion.
Light bosons only interact weakly with normal matter, making them extremely hard to detect. But it’s not impossible. There are two primary ways they could be found, and Huang and his colleagues are pursuing both. First, physicists could build sensitive experimental detectors to catch stray light bosons as they pass by Earth. And, second, astronomers could detect the signatures of light boson interactions in the wider universe, especially in the high energy density regions around black holes.
LAMPOST: A new light boson detector prototype
Recent advances in technology have made high-precision, low-cost detection experiments more feasible than ever before. One such experiment is a frisbee-sized detector called LAMPOST (Light A’ Multilayer Periodic Optical SNSPD Target), which is designed to search for dark photons. Developed by Huang, Perimeter Faculty member Asimina Arvanitaki, several former Perimeter postdoctoral researchers, and collaborators at MIT and the (US) National Institute of Standards and Technology, the prototype detector has already achieved a huge milestone by searching energy ranges no other detector has managed.
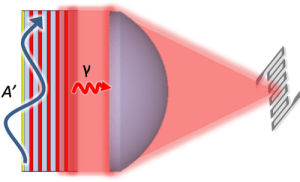
Dark photon interactions with normal matter are rare, but in some circumstances, through a coupling called “kinetic mixing,” they should be able to interact with – and transform into – regular photons, making them detectable. This transformation, called resonant conversion, doesn’t usually happen in nature, since photons travel fast (at relativistic speeds) while dark photons travel more slowly (at non-relativistic speeds). For the conversion to work, regular photons need to be slowed down.
LAMPOST relies on the idea that certain material structures can manipulate regular photons to make them “stop.” Unlike relativistic photons, these “stopped” photons, or “standing waves” of photons, can convert into dark particles. The idea is theoretically sound but practically challenging.
“Initially, we thought it was impossible to manufacture these structures,” says Huang. A happy accident changed that: during an unrelated visit to a colleague’s lab, he realized that the necessary material structures could be built fairly easily, with technology that didn’t exist a decade or two ago.
Another key to LAMPOST’s success is its extraordinary ability to remove background noise. “LAMPOST allows you to go to smaller wavelengths and higher energies. The experimentalists we work with, they designed these photon counters which are super precise, and they have almost no background. If you put it in a black box, and you wait, it might register a background photon every twenty-something hours. It’s so good,” says Huang. A normal camera sensor, by comparison, might register billions.
Last summer, the team reported only five background events in 180 running hours.
LAMPOST hasn’t found any new particles yet, but it is a compelling proof of concept. Now, it’s time to scale it up. There are new frequencies to scour for dark photons and different dark matter candidates to tease out.
“The LAMPOST structure can be used to look for any dark matter particle that is wave-like and not very heavy,” Huang says. “To look for different types of particles, the principle is the same, but you need different materials in the structure or, in the case of axions, you need a magnetic field.”
Looking for light bosons via multimessenger astronomy
A second way to look for light bosons is to scour the cosmos for them. Researchers suspect that in the early universe, the process of inflation could have produced light bosons in great quantities. They might also be produced in the extreme high energy density regions around black holes.
Huang and his cosmologist colleague, Perimeter Faculty member William East, recently examined how, through a process called superradiance, light bosons might gather in clouds and steal energy from the black hole.
In normal circumstances, energy cannot escape from a black hole, but superradiance takes advantage of a loophole: if black holes are spinning, then they have a large supply of rotational energy that can be extracted. When particles – or, more precisely, when waves (quantum physics tells us particles have properties that allow them to act like waves) – come near a spinning black hole, they can be scattered and amplified by the interaction. Through this process, small clouds of bosons around a black hole can grow exponentially: the larger the cloud, the faster it grows, until eventually it begins to leak energy out. These leaks release gravitational waves that might be detectable by Earth-based facilities, like LIGO (the Laser Interferometer Gravitational-Wave Observatory), or next generation gravitational wave detectors built in space. Gravitational wave astronomy may be the key that allows us to tease out light boson signatures around black holes.
But it’s not the only tool available, because light bosons can have different effects on different types of astronomical systems. In some instances, for example, a dark photon cloud produced via superradiance might emit not just gravitational waves but also a powerful detectable X-ray signal.
How does this happen? After all, X-rays are a product of normal electromagnetic fields, not dark electromagnetic fields. The answer is kinetic mixing: under these conditions, dark photons can interact with Standard Model particles that get caught up in the field, which emit regular photons. Through this process, a dark photon cloud can eventually produce a regular, detectable electromagnetic field. The final result is the creation of a magnetized plasma around the black hole that acts akin to the magnetosphere of a pulsar – a magnetized rotating neutron star.
Using modelling techniques learned from the study of pulsars, Huang and his colleagues determined that in such a system, the magnetic field lines would tangle up and reconnect, building energy in the system and ultimately releasing that energy in the form of powerful, periodic, and persistent X-rays and gamma rays. These emissions would be comparable to the brightest X-ray sources in the universe, meaning existing X-ray or gamma-ray telescopes could see them in distant galaxies hundreds of megaparsecs away.
One promising avenue of research, then, is to find ways to correlate gravitational wave signals in LIGO with electromagnetic emissions such as X-rays. Known as “multimessenger astronomy,” this process of combining data from multiple signal types could revolutionize the search for new particles.
The weird world of light bosons
The search for light bosons has already had its share of surprises. Just as Huang and East were able to take lessons from pulsar research to model light boson behaviour around black holes, they’ve also taken lessons from material science.
For example, when they examined the methods by which dark photons might be produced (either in the early universe, or around black holes) they discovered that newly created dark photons tend to turn into densely packed vortices – not dissimilar to the way vortices are created in type-II superconductors.
Superconductors are materials that, at very cold temperatures, can prevent a weak magnetic field from passing through them. This is known as the Meissner effect.
Type-I superconductors become non-superconducting when the magnetic field strength is raised above a certain threshold. But type-II superconductors enter an intermediate phase, in which tiny magnetic vortices – called Abrikosov vortices – form, allowing the magnetic field to pass through the material within the vortices. The higher the magnetic field strength, the more numerous the vortices become, until at last they become too densely packed and superconductivity is lost everywhere.
When dark photons are produced, they tend to turn into similarly structured vortices.
On the one hand, this is good news for researchers hunting for evidence of dark photons, as these vortex lines leave behind signatures. In the case of dark photon superradiance, for example, it opens up the possibility of a burst of energy release, known as a “bosenova,” when these vortex lines form. There are many exciting opportunities ahead for researchers to find dark photons via gravitational wave detectors or small-scale experiments.
On the other hand, the fact that dark photons tend to turn into vortices also probably means that dark photons don’t account for dark matter in the universe. It brings into question the longevity – or at least the ubiquity – of dark photon dark matter. In type-II superconductors, the Abrikosov vortices grab hold of the background magnetic field, and they contain almost all the energy density. “In the context of dark matter,” write Huang and East, “this would correspond to moving the energy that is stored in the background dark photon field into the vortices.” Those dense, energetic vortices subsequently radiate most of their energy away, either as dark photon radiation or as gravitational waves. In other words, the process depletes the supply of dark photon dark matter.
“It really makes us more inclined to switch to axion experiments,” says Huang. “We now question the original production mechanisms available for dark photons – it’s very hard to not get into a parameter space where all these vortex lines show up.”
That’s why it’s fortuitous that detectors like LAMPOST are versatile. By adjusting the material structures used (or by adding a magnetic field for axions), this type of detector can be tailored to search for a whole range of different particles.
Huang is confident that interdisciplinary efforts are the key to making progress. Bringing particle physics, material science, astrophysics, and cosmology together has already yielded surprising insights into the behaviour of light bosons, and there is so much more to discover.
Huang’s new Initiative for Small-Scale Experiment Design is intended to catalyze more innovative and adaptable experiments by enabling collaboration between multidisciplinary theorists and experimentalists.
“You’ve got to open dialogue to make breakthroughs,” says Huang. “We want to get everyone in the same room and see what’s possible.”
The LAMPOST team includes Jeff Chiles, Ilya Charaev, Robert Lasenby, Masha Baryakhtar, Junwu Huang, Alexana Roshko, George Burton, Marco Colangelo, Ken Van Tilburg, Asimina Arvanitaki, Sae Woo Nam, and Karl K. Berggren. Phys. Rev. Lett. (2022) 128.
William East and Junwu Huang’s dark photon vortex research is published in JHEP (2022) 089.
The research describing multimessenger techniques to find light bosons that have caused pulsar-like X-ray emissions around black holes was carried out by Nils Siemonsen, Cristina Mondino, Daniel Egana-Ugrinovic, Junwu Huang, Masha Baryakhtar, and William East. Phys. Rev. D 107 (2023) 075025.
À propos de l’IP
L'Institut Périmètre est le plus grand centre de recherche en physique théorique au monde. Fondé en 1999, cet institut indépendant vise à favoriser les percées dans la compréhension fondamentale de notre univers, des plus infimes particules au cosmos tout entier. Les recherches effectuées à l’Institut Périmètre reposent sur l'idée que la science fondamentale fait progresser le savoir humain et catalyse l'innovation, et que la physique théorique d'aujourd'hui est la technologie de demain. Situé dans la région de Waterloo, cet établissement sans but lucratif met de l'avant un partenariat public-privé unique en son genre avec entre autres les gouvernements de l'Ontario et du Canada. Il facilite la recherche de pointe, forme la prochaine génération de pionniers de la science et communique le pouvoir de la physique grâce à des programmes primés d'éducation et de vulgarisation.