“A lot of people take the idea that we want to build a quantum computer for granted,” says Roger Melko. “They have obvious applications. But we forget that computers also drive fundamental discovery in physics, in ways that are surprising and significant.”
Surprising and significant fundamental discoveries driven by computers are Melko’s stock-in-trade.
Melko is a Perimeter associate faculty member, cross-appointed with the University of Waterloo (UW), whose research involves using simulations to understand the exotic states of matter where quantum properties are important. For instance, he and Perimeter postdoc Juan Carrasquilla discovered that the same kind of machine learning that can sort photos of cumulous clouds from photos of cauliflower can also tell the difference between different states of quantum matter – even states that advanced computers can’t simulate.
He’s also turned his group’s expertise in numerical simulations towards understanding quantum computers themselves. Recently, in simulations of how a quantum circuit should work, they glimpsed something unusual: a new type of phase transition.
What did they find, exactly? “It’s called a measurement-induced phase transition,” Melko says. “I think it can only really occur in a quantum computer.”
In other words, it’s brand new physics.
About phase transitions
Phase transitions are everywhere in physics – popping up every time a system has more than one way to behave and the shift from state A to state B is sharp.
For instance: put a block of ice in a warm room. At first, a thermometer stuck into the ice shows the ice warming slowly. At the melting point, though, something strange happens: the thermometer stalls out, even though heat is still being absorbed. All that heat is driving the change of state, from solid to liquid. Only after all the ice is melted will the thermometer, now sitting in a puddle, start to move again.
Two ways to behave and a sharp point of transition between them: that’s the basic definition of a phase transition. There are many other examples: Graphite shifting into diamond as pressure is added is a phase transition. A fridge magnet losing its magnetism when hit by a hammer is a phase transition. Even the moment when the early universe changed from a charged plasma to neutral hydrogen was a phase transition.
The measurement-induced phase transition spotted by Melko and his team looks enough like these phenomena that it’s recognizable as a phase transition. But some of the details don’t line up with any known physics.
The team’s simulations, Melko explains, are state-of-the-art digital replicas of a quantum computer’s circuit board. The circuit can have two different kinds of behaviour: one in which entanglement grows rapidly, and one in which entanglement grows significantly more slowly. The transition between the two kinds of behaviour isn’t gradual, it’s sudden: a phase transition.
“It looks almost identical to a phase transition in matter,” says Melko. “That’s the first part that’s amazing. You have this really non-trivial quantum circuit that’s built in the lab – it has nothing to do with matter or materials or so on – yet it has a phase transition that looks like a phase transition in matter.
“The second part that’s amazing is that even though it looks like something we would see in matter, there are some things we don’t understand about it. It’s clearly a new kind of phase transition that doesn’t seem to occur in the natural world.”
Universality classes, or why a new kind of phase transition is a big deal
A new kind of phase transition is a big deal.
To explain why, Melko lifts his glass of water into the sunlight. “If I look at the phase transition between water and vapour – if I heat this glass of water up – it will have the same properties as a magnet going through a phase transition in the state of its magnetic field. Or those phase transitions in the early universe. That’s called universality. I think it’s the deepest thing in physics.”
Universality is a mathematical signature. In much the same way that all things based on circles will be described somewhere along the line with the number pi, all known phase transitions are described with just a few constants. Like pi, these constants are ratios: measure something both before the transition and after, compare the two, and watch these familiar constants emerge.
There are just a handful of patterns to the way these constants occur again and again in different kinds of phase transitions. One class of phase transitions might involve alpha, beta, and gamma, for instance. Another class, beta, gamma, and kappa. Each of these sets of constants is called a universality class.
Physicists have a pretty good, though not perfect, handle on universality classes, says Melko. “We understand which systems display which kinds of universal behaviour. We understand what the fundamental underlying reasons are that this phase transition has this universality class while that phase transition gives us that universality class – we believe we understand all that.”
Remember that Melko’s simulation showed that a simple quantum circuit could switch suddenly from one mode of behaviour to another – undergoing a phase transition. That was surprising by itself. But what was more than surprising – verging on shocking – was that this phase transition didn’t fit any defined universality class.
Deep in a simulation of an unbuilt quantum computer, Melko had glimpsed new physics.
Measurement-induced phase transitions in detail – and in the wild
The team’s paper, Melko says, is a proposal to build the circuit and study the phase transition in the wild. The theorists are working closely with Rajibul Islam at the Institute for Quantum Computing to make it happen. Islam has built a “trapped ion” quantum computer, where the qubits are single atoms trapped in electromagnetic wells.
“We sat down one day and said: Do you think we could build one of these phase transitions inside Rajibul’s computer? Is that even possible? That’s what this paper is. It’s a numerical study, where we used state-of-the-art computational libraries to simulate Rajibul’s computer.”
Melko sketches out a line of qubits – each would be one of the trapped ions in Islam’s setup. These qubits are operated on by a series of gates and become entangled. After this operation, they reach an end state, where they can be measured. This process, all together, is a simple quantum circuit.
Then Melko adds a layer of complication: at each step through the gates, he can add a measurement of one or more of the trapped atoms. This measurement kills the entanglement. “It’s like checking to see whether the cat is alive or dead,” he says, referencing Schrödinger’s famous thought experiment. “The measurements force the qubit into a well-defined state, and quantum effects like entanglement wash away.”
Depending on the number of local measurements, the quantum circuit has two modes of behaviour: one that grows entanglement quickly, and one that doesn’t. A circuit with less measurement has more entanglement in its end state.
But the relationship isn’t linear: the circuit seems to be able to handle a few measurements with only a small fall-off in entanglement. But inch past the critical measurement threshold and the entanglement dives from a cube to a square. Graph it, and it looks for all the world like something melting. It’s definitely a phase transition: a measurement-induced phase transition.
The upshot? “Yes, a trapped ion quantum computer can be used to realize this kind of phase transition.”
Such is the promise of this new discovery that researchers around the globe have seized on it. Just at Perimeter, there are three researchers working on the topic independently.
Faculty member Beni Yoshida is attempting to better decode entanglement structure in these circuits. This very new work is available in pre-print only.
Faculty member Timothy Hsieh was also interested. Hsieh, who recently received a prestigious Early Researcher Award from the Ontario Ministry of Colleges and Universities, is interested in quantum materials broadly, and particularly in artificially created quantum materials, which give researchers more control and power over quantum effects and better ways of encoding quantum information. This new phase transition was exactly up his alley.
Working with PhD student Shengqi Sang, Hsieh leveraged the new kind of phase transition as a new tool to explore certain interesting but difficult-to-study phases of matter.
“I decided to look at the phase in which the measurements dominate and give rise to a low-entanglement final state,” he says. Usually, measurement erases effects like entanglement and superposition: open the box, and Schrödinger’s Cat is no longer in alive/dead superposition: kitty is now either fully alive or fully dead.
But that’s not what’s happening in this quantum circuit, where even the post-measurement phase contains long-range quantum correlations. In fact, says Hsieh, it’s even more interesting than that. The structure of the measurements you make during the phase transition helps preserve and control these long-range correlations.
He calls the low-entanglement phases of the quantum circuit “measurement-protected quantum phases.” They belong to a class of phases known as dynamical phases or non-equilibrium phases. Most states describe structure; dynamical states describe behaviour. Think of describing a clock, for example: the most salient thing isn’t the length of the hands or the layout of the numbers (the structure) but the fact that the hands move at a particular speed (the behaviour).
Dynamical phases are important, but they’re challenging for physicists. “Over the last decade we’ve come to understand equilibrium phases pretty well,” Hsieh says. “But dynamical phases – phases that are out of equilibrium – that’s the Wild West. We don’t have the law out there yet.”
Structuring the measurements in the phase transition gives Hsieh a new tool to explore the wild land of non-equilibrium phases. The newly opened line of study is attracting interest from quantum matter researchers around the world.
Quantum computers as telescopes
That more than one group is on the track of discovering new physics hiding inside early quantum computers is not too surprising, says Melko. “People are starting to catch on to the idea that the quantum computers we have today – or maybe the quantum computers we have next year; the nearest of near-term stuff – could be able to realize new physics,” he says.
“We’re on the verge of discovering a new phenomenon in the laboratory that we never really thought to look for and that really tests our understanding of quantum field theory. This is a quantum computer as an instrument for discovery – like a new telescope or a new particle accelerator or a new gravitational wave detector.”
With all the focus on the undeniable commercial potential of quantum computers, he says, we sometimes lose sight of this aspect of discovery.
Consider this. After hearing about the Dutch “perspective glasses” in 1609, Galileo improved on the ideas and constructed his own telescope. One of the first things he did with it was demonstrate it to the leaders of Venice, who used it as a spyglass to give tactical advantages to their seagoing state.
Galileo himself, though, turned the telescope upward toward the “perfect” heavenly spheres, discovering that the moon was not a perfect sphere, that the sun had spots, and that Jupiter had moons of its own. The new way of looking at the heavens shifted our entire view of what the heavens were.
Quantum computers could be the same. The tactical advantages they might give are huge and just over the horizon. But the new view they give us on the quantum world is opening right now.
Further exploration
- Dive deeper: Read Melko and co.'s Physical Review A paper
- Dive deeper: Read Melko and co.'s Physical Review A paper
- Read more: Check out Timothy Hsieh's paper on "Measurement-protected quantum phases"
- PIQuIL: Learn more about the Perimeter Institute Quantum Intelligence Lab
- People of PI: Quantum creator Timothy Hsieh
About PI
Perimeter Institute is the world’s largest research hub devoted to theoretical physics. The independent Institute was founded in 1999 to foster breakthroughs in the fundamental understanding of our universe, from the smallest particles to the entire cosmos. Research at Perimeter is motivated by the understanding that fundamental science advances human knowledge and catalyzes innovation, and that today’s theoretical physics is tomorrow’s technology. Located in the Region of Waterloo, the not-for-profit Institute is a unique public-private endeavour, including the Governments of Ontario and Canada, that enables cutting-edge research, trains the next generation of scientific pioneers, and shares the power of physics through award-winning educational outreach and public engagement.
You might be interested in
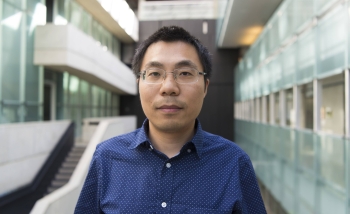

