On the shoulders of Bell
A lot of things got shaken up in 1964. The Beatles took America by storm, Cassius Clay became Muhammad Ali (and the heavyweight champion of the world), and a physicist from Northern Ireland named John Stewart Bell showed that reality was not as it seemed.
Bell showed that quantum mechanics allowed for weird correlations between two specially prepared, widely separated systems – correlations that couldn’t be explained in terms of “hidden variables” that led to the outcomes observed at the two locations in a straightforward, deterministic way. Such systems are said to be “entangled,” meaning that a measurement of one member of the pair immediately yields information about the second member, regardless of how far away it may be.
This apparent connection between spatially separated systems had been foreseen by Einstein some 40 years earlier, and he wasn’t happy about it. Physics as it was then understood had been founded on “local” interactions – for A to influence B, A has to be near B – rather than non-local influences, which Einstein derided as “spooky action at a distance.” Nonetheless, numerous experiments from the 1980s onwards have shown that these weird correlations are real.
Now, a team of scientists led by Elie Wolfe, a research associate at Perimeter Institute, has taken Bell’s theorem a step further. They have shown not only that nature is non-local but that this non-locality is inherently “multipartite” – that is, involving multiple parties, not just pairs of systems.
Not only that, Wolfe and his colleagues have shown that this is the case even if quantum mechanics is replaced by some broader, more general theory. In other words, no matter how well you understand the physics of every possible pair of entangled particles, it’s not enough: Some correlations can only be explained by examining three-way entangled systems, four-way entangled systems, and so on.
It’s a remarkable result – and one that could only be achieved by standing on Bell’s shoulders, so to speak.
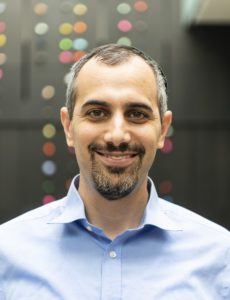
“Bell’s theorem was shocking,” says Wolfe. He describes it as a “major paradigm shift” because it allowed physicists to rule out a certain class of hidden variable theories – that is, theories that would “save” locality by explaining the strange correlations seen in entangled systems as the result of hidden variables that describe conventional, local interactions.
Bell’s theory pulled the plug on those simple hidden variable models – and Wolfe and colleagues’ new work rejects a class of theories broader than all hidden variable theories, leading to the conclusion that “nature’s nonlocality must be boundlessly multipartite,” as Wolfe wrote in a recent paper. (A companion paper focuses specifically on the failure of solely bipartite connections.)
As is often the case with quantum mechanics, the result is deeply counterintuitive; there simply isn’t anything in the classical world that’s quite analogous.
Still, it is perhaps useful to think of a cartoonish analogy, even if it does nothing more than introduce the flavour of the problem. Suppose it’s the early 1960s: You’re George Martin, the Beatles’ producer, and you’re wondering what sort of songs the band’s three songwriters, John, Paul, and George, are likely to come up with. (For the purposes of this not-great analogy, we’ll ignore poor Ringo, as catchy as “Octopus’s Garden” would turn out to be.)
First, you think, “Well, I know what each of them can do individually – surely that’s enough.” But it’s not – because you quickly discover that when any two of them get together, the results are very different from what you’d get by simply combining the individual work of each member of the pair. Then you think, “Okay, I need to know what each pair of them is likely to produce” – and so you study what happens when John and Paul collaborate, and when John and George collaborate, and when Paul and George collaborate.
And yet, this still doesn’t do the trick: No matter how well you understand each of the pairs, something different happens when you put all three of them into a room and ask them to make music. Again, it’s a very loose analogy – people are not quantum systems – but it perhaps gives the flavour of what Wolfe and his colleagues have discovered about the quantum world.
Actually, the result goes beyond mere quantum mechanics: Wolfe and his team have been exploring a broad class of theories known as generalized probabilistic theories, or GPTs. This broad class of theories “tries to situate quantum theory as one of an infinite spectrum of possible theories,” he says.
What all these theories have in common is that they go beyond hidden variable explanations; they further allow for exotic manners of generating statistical correlations among physical systems. “It turns out our universe fits a description in terms of quantum theory,” Wolfe says, “but we can imagine more exotic theories also being plausible descriptions.”
The result may shed some light on what quantum theory is actually telling us about the world, says Jonathan Barrett, a physicist at the University of Oxford (and a Perimeter Visiting Fellow). It was already clear that the theory seems to require a universe built on non-locality; this research helps pinpoint exactly what kind of non-locality is needed.
“What they show in this paper is that, essentially, for any theory that would allow this pair-wise non-locality, no matter how strong that pair-wise non-locality is, it will never be able to recover quantum predictions that you can get from a source of three particles,” says Barrett. “Quantum non-locality, then, has a genuine three-way nature to it.”
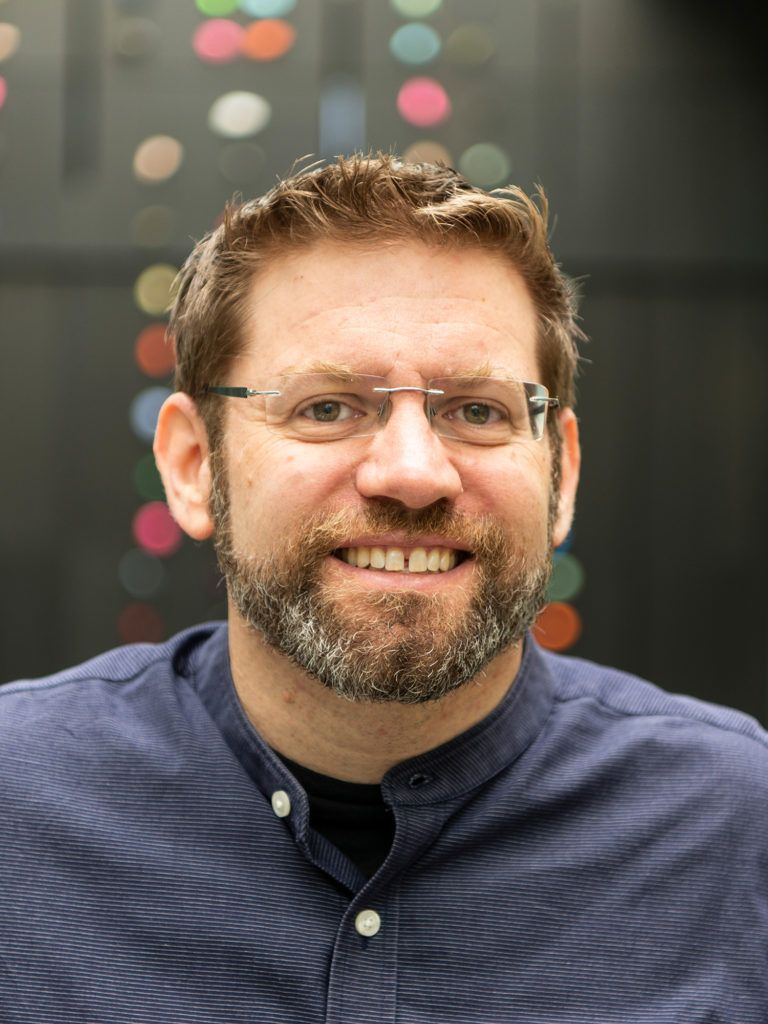
Wolfe examined this question – whether the outcomes of all possible measurements can be explained in terms of entangled pairs – not only from the point of view of quantum theory but from the perspective of these broader alternative theories. And it turns out that “there’s some intrinsically three-way non-classicality, even if you make the two-way non-classicality as extreme as you like,” says Perimeter Faculty member Robert Spekkens, a colleague of Wolfe’s.
No matter how much we “soup up” the interactions between pairs of systems, they can only explain so much. “There are still some three-way correlations that can’t be explained,” says Spekkens. And, as the new work shows, even ramping things up beyond quantum theory and into the realm of the GPTs isn’t enough; these three-way correlations still can’t be explained.
In other words, Bell’s theory seemed to pave the way for non-locality, and we now find that this non-locality rests on even stronger footing. Bell’s theorem is important, Spekkens says, because it challenges the notion that correlations should have straightforward causal explanations.
The simplest way to try to explain the kinds of correlations seen in a quantum entanglement experiment – say, one involving the spin of an electron at A and the spin of another electron at B – is to propose that some set of hidden variables ultimately determined the outcomes of measurements at both A and B. (A classic example involves a pair of gloves: If one glove is sent to Alice and the other to Bob, when Alice discovers that she has, say, a “left” glove, she knows right away that Bob has a “right” glove.)
That would be “a very natural guess” for a causal explanation in that sort of experiment, Spekkens says – but then Bell comes along, and that simple hidden variable model goes out the window. “And so you’re in this uncomfortable position of asking, ‘Oh, do I have to give up on the idea of having a causal explanation of correlations? Or is it just that I have to innovate the notion of what a causal explanation is?’”
“I personally think it’s the latter,” says Spekkens. “But there are some people out there who think that we have to be ‘anti-realist’; we have to just say, there are some correlations that don’t get explained causally.” (Anti-realist, in this context, refers to the idea that all a physical theory needs to do is to account for observations, even if it fails to describe what the universe is “really” made of.)
The reason Spekkens prefers to stick with causal explanations is that causality underlies so many of our basic assumptions about the physical world. Causal explanations are “really the essence of science,” he says.
We end up with this perspective, he suggests, just by thinking about everyday cause-and-effect happenings.
“If anybody in a scientific field with statistical data were to say, ‘I’ve seen this correlation between a certain activity and certain health benefits,’ and they don’t answer the question ‘Was it a common cause, or was it a cause-effect relation?’, then they haven’t really made the case for doing that activity. Correlation does not imply causation; you need to establish that it’s a cause-effect relation, not merely a common cause,” says Spekkens.
“Statistical correlations are not really the thing that we’re looking for, in science. We’re looking for cause-effect relations. To really understand what’s going on in a system is to understand it causally.”
That’s why the work of Wolfe and his colleagues is so intriguing, Spekkens says: “Anytime we have a Bell-like result, it says: ‘Here’s a challenge to causal explanations.’ And that’s a great clue as to how the quantum world is different from the classical world."
Another interesting aspect of this work is that is seems to mesh with recent results from a completely different branch of science – machine learning – and, in particular, a field known as “causal inference,” which tries to tease out causes and effects from data sets.
Of course, cause and effect lie at the heart of many kinds of scientific problems. Consider the correlations seen between smoking and cancer. Do the correlations by themselves prove that smoking does in fact cause cancer? Decades ago, a tobacco company might have argued that some third factor acted as a “common cause” of both smoking and cancer – say, a genetic trait that made one more likely to take up smoking and which also made one more likely to get cancer.
Such a factor, if it existed, would be something like the hidden variables that theorists suggested might explain away the indeterminism seen in quantum mechanics. But Bell’s theorem ruled out a certain class of hidden variable theories (namely “local” hidden variable theories, which preserve locality).
“It turns out that, in retrospect, what Bell was doing in the 1960s is very well classified as progress in the field of causal inference,” says Spekkens.
The equations that Bell spelled out in 1964, known as Bell inequalities, act as constraints on the statistical distribution that can be observed when measuring correlations between pairs of classical variables.
In the lab, if we see those constraints violated, Spekkens says, “then you know the causal structure is not just, ‘Hey, there’s a common cause leading to the outcomes, and settings that influence the outcomes.’ And so you have to go looking for some other causal explanation.”
Spekkens notes that these two separate groups of scientists – those working on machine learning and those working on quantum physics – latched on to the same terminology. “The causal inference community – who had nothing to do with quantum physics – also used this term, ‘hidden variables,’ or ‘latent variables.’”
GPTs take this idea further, allowing us to imagine scenarios where there are even more extreme violations of the Bell inequalities. These broader theories are “ways the world could have been, if it wasn’t quantum,” says Spekkens. “And that framework allows us to talk about correlations that are even stronger than what we see in quantum theory. So, we can imagine these other worlds where we would see more Bell inequality violations.”
And yet, as this new work shows, even in a world that allowed for these stronger correlations, you “still don’t get everything,” as Spekkens puts it – that is, the correlations seen among trios of particles can’t be predicted by examining all the correlations among all the pairs of particles. All of which means that physicists are getting a deeper understanding of cause and effect, even without committing to any one theory (because GPTs encompass a whole array of possible theories).
“And that’s really interesting, that you can make these strong conclusions about causal structure, even though you’re making almost no assumptions about the nature of the physical theory,” says Spekkens.
While such matters may seem abstruse and theoretical, one particular branch of quantum physics, involving quantum information, has already led to remarkable new technologies, including whole new industries focused on quantum telecommunications and quantum cryptography. In fact, Wolfe refers to the kinds of correlations seen in quantum physics experiments as “resources” that can be exploited. (An article published last year by Wolfe, Spekkens, and three co-authors explored this idea in detail.)
“Entanglement is a resource that’s available in nature, like water and oil and electricity, and we can do all kinds of things with it,” says Wolfe. “And it turns out that three-way entanglement is its own unique resource.”
Meanwhile, the effort to make sense of quantum theory – an effort that now has more than a century of theorizing under its belt – continues. While quantum theory has been astoundingly successful, “it isn’t really predicated on a physical model, the way that other physical theories are,” says Wolfe. “And so it’s a little harder to be confident that it’s correct.” As the research into GPTs suggests, quantum theory is one of many ways that the universe “could have been.”
“You can picture quantum theory as a sort of point in a great space of possible theories,” says Barrett. “And for whatever reason, our universe has chosen that point, to be the theory that describes it. And the others are, as far as we know, sort of fictional. But you can still ask, ‘Why did nature choose that point, and not any of the others?’”
Of course, the theory works; it has been tested over and over again, often with fantastic precision. Even so, that leaves open the possibility that “it might not be the final description of reality,” says Wolfe. Still, he believes that “we’re coming to understand that quantum theory is ‘more right’ than we thought. It comes with a lot of philosophical baggage – but evidently much of that baggage is indispensable.”
Postscript: Wolfe's work has recently been followed up experimentally by three separate collaborations. Technically-minded minded readers can look up the papers on the arXiv preprint server at: https://arxiv.org/abs/2201.12754, https://arxiv.org/abs/2201.12753, and https://arxiv.org/abs/2203.00889.
---------------
Dan Falk (@danfalk) is a science journalist based in Toronto. His books include The Science of Shakespeare and In Search of Time.
Further exploration
About PI
Perimeter Institute is the world’s largest research hub devoted to theoretical physics. The independent Institute was founded in 1999 to foster breakthroughs in the fundamental understanding of our universe, from the smallest particles to the entire cosmos. Research at Perimeter is motivated by the understanding that fundamental science advances human knowledge and catalyzes innovation, and that today’s theoretical physics is tomorrow’s technology. Located in the Region of Waterloo, the not-for-profit Institute is a unique public-private endeavour, including the Governments of Ontario and Canada, that enables cutting-edge research, trains the next generation of scientific pioneers, and shares the power of physics through award-winning educational outreach and public engagement.