Searching for axions
In 1880, the French brothers Pierre and Jacques Curie demonstrated a remarkable and unusual property of nature.
When certain types of materials – such as quartz, tourmaline, topaz, cane sugar, or Rochelle salt – are compressed or squeezed, an electrical charge is generated across the material.
This is piezoelectricity, derived from the Greek word piezein, meaning to squeeze or press.
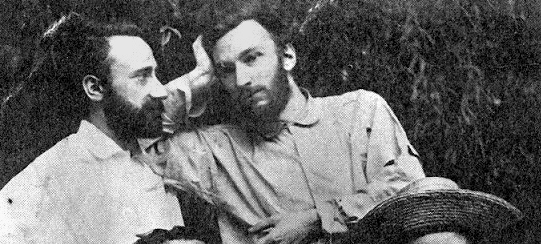
Pierre (who later married Marie Skłodowska, better known as Marie Curie, and shared the Nobel Prize with her for their work on radioactivity) and Jacques’ demonstration turned out to be incredibly useful.
Its applications have included electronically amplified guitars and electronic drums, clock generators, and scanning probe microscopes that resolve images at the scale of atoms.
Now, a collaboration between Asimina Arvanitaki, a particle physicist from Greece who holds the Stavros Niarchos Foundation Aristarchus Chair at Perimeter; Amalia Madden, a recently graduated Perimeter PhD student; and Ken Van Tilburg, a joint faculty member at New York University and the Flatiron Institute, is proposing that the same effect might also be used to probe for hypothetical dark matter particles known as axions.
They have a recently published a paper titled “Piezoaxionic effect” in Physical Review D. In it, they put forth the possibility that in some piezoelectric materials, axions could produce a detectable signal, which they call the “piezoaxionic effect.”
Arvanitaki is well-known for designing innovative “tabletop” experiments that are much smaller – and a fraction of the cost – of giant particle accelerators, yet still extremely precise. These experiments could deliver data that might crack open some fundamental questions in physics.
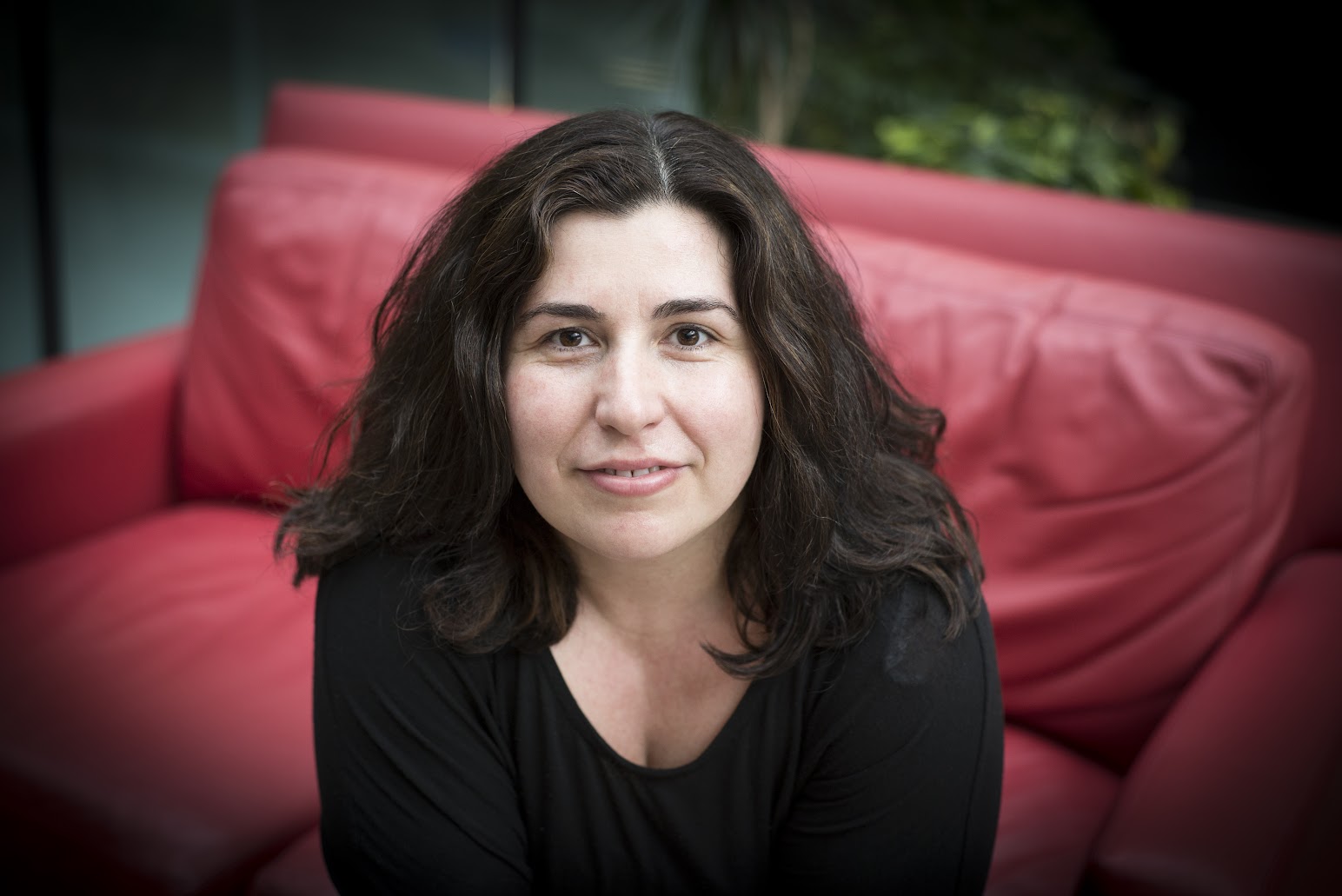
This idea of probing for axions via the piezoelectric effect “falls in the category of tabletop experiments that have great sensitivity,” Arvanitaki says.
Next, they’ll need to get an experimental group to test out the idea.
To probe for these effects, experimentalists could use much of the same technology that was proposed in previous research published in Physical Review Letters, titled “Sound of Dark Matter: Searching for Light Scalars with Resonant-Mass Detectors.” That research explored the idea that dark matter could be a type of wave that resonates, like a guitar string.
The scientists are suggesting that that the axion background has a kind of oscillating “breathing” effect. If so, then this axion background can produce an electric displacement field that can give rise to a voltage across a crystal. They call this the “electroaxionic effect.”
An important part of the axion story has to do with what is known as “symmetry breaking.”
Symmetry is an inherent aspect of nature that refers to properties of a physical system that remain the same no matter how the rest of the system changes.
There are different types of symmetries in physics. Spatial translation symmetry, for example, means that certain laws of physics (such as the speed of light, but other laws as well) are the same here, in Australia, on the moon, and presumably even in some far away corner of the universe.
But there are other types of symmetries that are important to underlying laws governing the behaviour of particles and forces. Charge symmetry, for example, allows positively charged particles to be swapped for negative ones without changing the behaviour of the overall system.
Equally beautiful is the fact that symmetries can be broken, which can also lead to new and yet-undiscovered aspects of nature.
One can imagine symmetry to be like a perfectly balanced pencil standing on its tip – a symmetrical system. When it falls, tipping over in one direction, the symmetry is broken. Something new has happened.
Symmetry breaking can result in new particles and forces being revealed. The existence of the quark, for example, was predicted thanks to an observation of broken symmetry. Also, the Higgs mechanism that gives mass to particles relies on symmetry breaking. When the universe was born, the Higgs field might have been in a nicely balanced, symmetrical state, like the pencil standing on its tip. But fractions of a second later, it tipped over.
Likewise, in certain types of piezoelectric crystals, the breaking of other types of symmetries – namely parity symmetry and time-reversal symmetry – can provide a window into the existence of the axion field. “These broken symmetries are what make these crystals so well matched to the search for the axion field,” Madden says.
Parity symmetry is the idea that the laws of physics should be the same if a particle is swapped with its antiparticle and it is inverted or mirrored. Time-reversal symmetry is the idea that the laws of physics should be the same whether you look at a system going forward or backward in time.
It was Chien-Shiung Wu, also known as Madam Wu or the “First Lady of Physics,” who produced a beautiful experiment that demonstrated that beta decay, which is governed by the weak force, can violate parity symmetry.
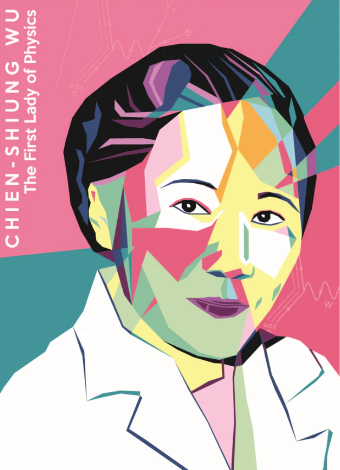
But this is odd because both charge and parity (CP) symmetries are preserved in the strong force that keeps the quarks and gluons tightly bound in the nuclei of atoms. The Standard Model of particle physics cannot easily explain why strong force interactions do not violate the charge-parity symmetries while the weak force interactions can. This is the “strong CP” conundrum in physics.
So, besides being good dark matter candidates, the axions, if discovered, could help solve the strong CP problem.
Madden adds that the axions themselves are doing the work.
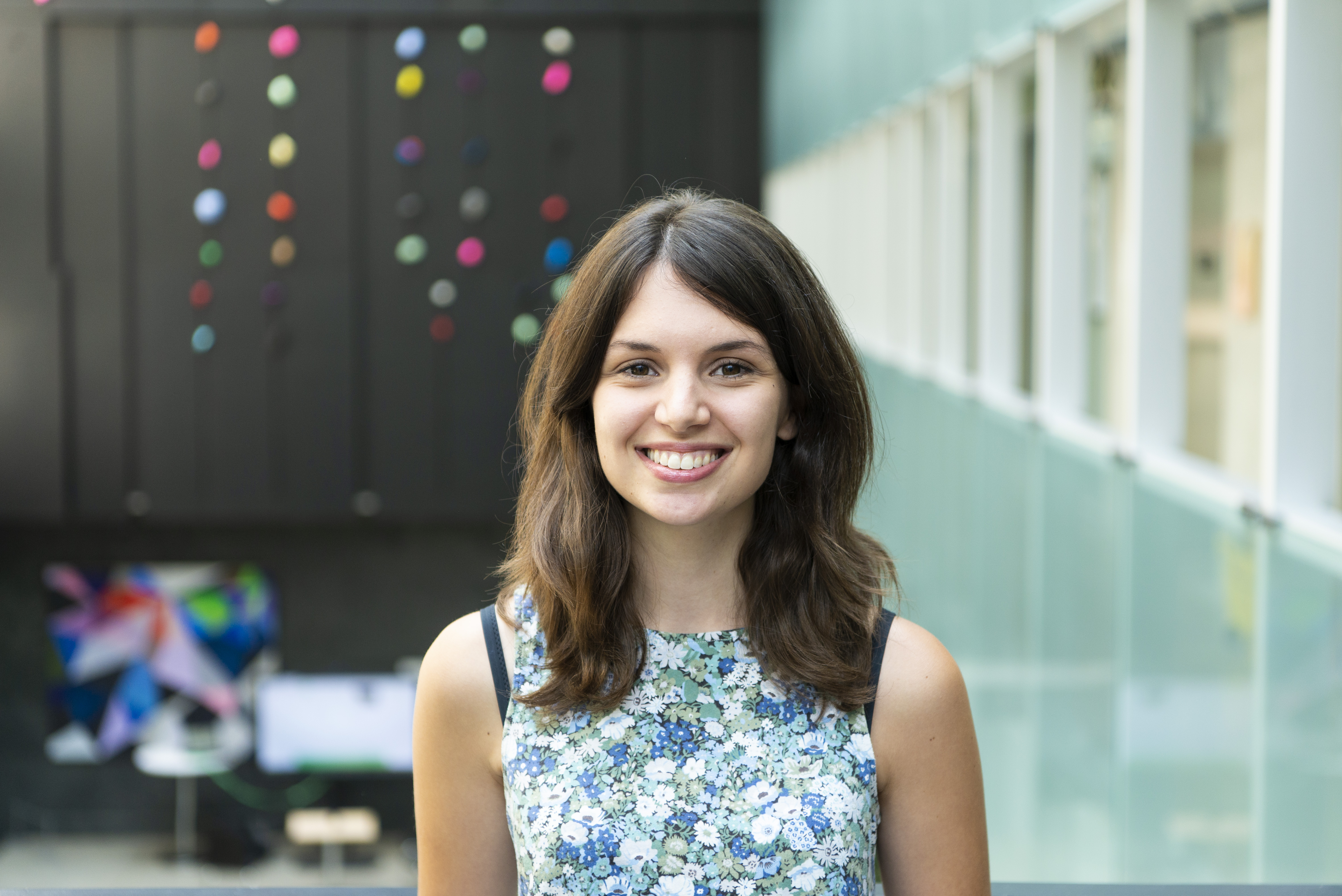
“The axions are already oscillating or breathing,” she says. “But because of the nature of piezoelectric materials when you deform them, you could get this electric current or electric signal and then we can read out that signal.”
Physicists around the world are eager to find a way to detect axions. This new paper provides a fresh idea that the researchers hope will gain traction with experimentalists.
Detecting such signals will not be easy. “We are looking for a really tiny signal,” Madden says. She adds that the first step toward building an experiment will be identifying the type of crystal or material where these effects are most likely to be detected.
“It is not just any piezoelectric crystal that will work, because we need these special types of nuclei. Once experimentalists have made a very, very pure crystal for us, then it needs to be cooled down to a very low temperature,” Madden says. “This is not something you can do in your basement. You need specialized equipment.”
Despite the challenges, it is not an unrealistic idea. There is equipment today that can measure extremely tiny electrical currents. By running the experiment over a long period of time and gathering a lot of data, it might be possible to find the axion signatures, Madden says.
Much of the technology has already been developed in looking for gravitational waves with resonant mass detectors, Arvanitaki adds. “The hardest part of what we are proposing is identifying the right material to test,” she explains. “We need materials with atoms that have very heavy nuclei. But at the same time, we need them to have low radioactivity because we need to put them in a very cold environment.”
Arvanitaki adds that discovering the effects of axions would be a wonderful addition to the piezoelectric effect story that has a long history and is connected to work that the famous Curie brothers were doing 144 years ago.
The nature of dark matter has been a mystery for decades and there is no guarantee that future experiments will find the signals proposed in this recent paper.
But Arvanitaki says the best that theoretical physicists can do is make educated proposals that can be tested in future experiments. “We think that’s what we have done in this paper,” she says. “The axion is a well-motivated particle; it has a reason to be there. But what nature does, that is a whole different story. It has no obligation to follow what we say.”
For Madden, the chance that a detection could be made at all is exhilarating. “It would be phenomenal,” she says. “I do not think we can overstate how exciting that would be. But even if they do not find the signal, if somebody builds the experiment, we would be thrilled.”
About PI
Perimeter Institute is the world’s largest research hub devoted to theoretical physics. The independent Institute was founded in 1999 to foster breakthroughs in the fundamental understanding of our universe, from the smallest particles to the entire cosmos. Research at Perimeter is motivated by the understanding that fundamental science advances human knowledge and catalyzes innovation, and that today’s theoretical physics is tomorrow’s technology. Located in the Region of Waterloo, the not-for-profit Institute is a unique public-private endeavour, including the Governments of Ontario and Canada, that enables cutting-edge research, trains the next generation of scientific pioneers, and shares the power of physics through award-winning educational outreach and public engagement.