Eight ways physics has changed since you were in high school
Do you remember the days when dinosaurs didn’t have feathers and Pluto was a planet? It’s all out-of-date science now, and there’s a certain nostalgia attached to those memories.
But keeping up with the latest discoveries gets us asking bigger and better questions. So if you’ve been out of the loop for a few decades, it’s time to brush up. Here are some ways physics has changed since you were in high school.
1. The universe probably won’t end in a big crunch.
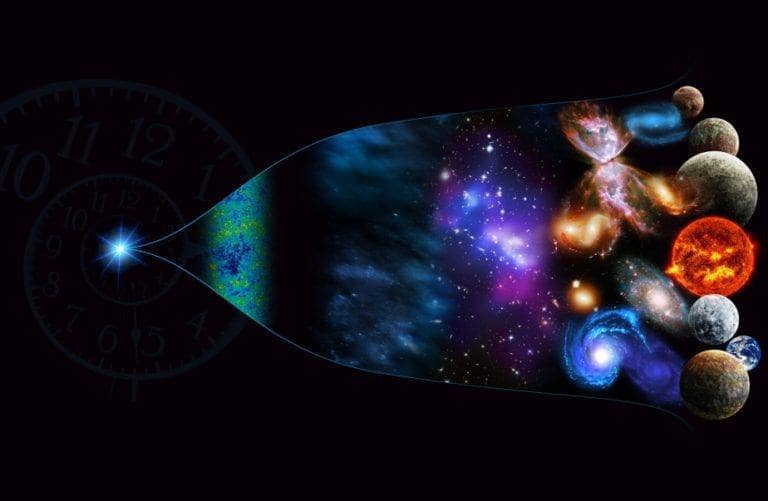
Scientists have speculated about the end of the universe for years, and we have now ruled out, with reasonable certainty, one possible fate. The Big Crunch, in which gravity squeezes the universe back into a singularity, was a leading theory for most of the 20th century. Not anymore. The discovery of dark energy – an energy source first observed in 1998 – told us the expansion of the universe is accelerating. Gravity won’t be strong enough to reverse it.
Today, most cosmologists expect the universe to expand forever. In this scenario, all the usable energy in the universe eventually dissipates in what is called the Big Freeze (or “heat death”).
Cosmic annihilation might come in other forms too: If the expansionary effects of dark energy increase rather than stay constant, it could pull the universe apart at the atomic level, in an event called the Big Rip.
Or, the universe might just fall apart, due to a phenomenon called false vacuum decay.
Vacuum decay is unique among these scenarios in that it’s unobservable: A near-immortal being could watch the universe’s heat death (which would be very boring) or witness the Big Crunch approaching (which would be scary). But it would not see vacuum decay coming, because the decay spreads at the speed of light.
If the final fate of the universe keeps you up at night, you’re not alone, but at least rest easy knowing that the Big Crunch isn’t likely. You can channel your existential dread elsewhere.
2. The cosmological constant might not be constant.
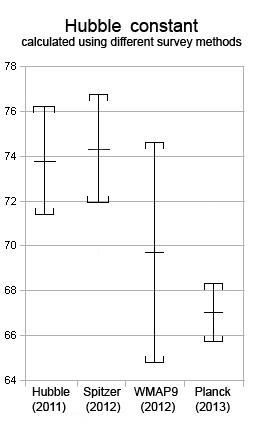
Primefac, CC BY-SA 3.0 [/caption]
Even after discrediting the Big Crunch, the accelerating expansion of the universe hasn’t finished rewriting physics textbooks. In the last few years, it’s spawned a new astronomical mystery. Dramatically dubbed the “crisis in cosmology,” it has physicists the world over scratching their heads.
In actual fact, it’s less a crisis and more a puzzle that has researchers excited. If we solve it, it might just help fill some of the gaps in our understanding of the universe. Here’s what you need to know:
The cosmological (or Hubble) constant is a measurement of how fast galaxies are moving away from us: the further away they are, the faster they seem to move. It tells us the universe is expanding, and the expansion is accelerating.
Theorists expected the Hubble constant to be a single, unchanging number. But the universe seems to say otherwise. There are a couple of ways to measure the constant, and each gives different answers – not even within one another’s margin of error.
Measurements using the cosmic microwave background radiation – the remnant light of the early universe, imaged by the European Space Agency’s Planck spacecraft between 2009 and 2013 – give a rate of about 67.7 (km/s)/Mpc. Meanwhile, measurements using type 1a supernovas (which have a standard brightness, making distance measurements between them wonderfully precise) give 73 (km/s)/Mpc. Astronomers have devised several alternative measurement methods, but none resolve the tension.
Is one of the measurements wrong? Maybe, but they’ve all been triple-checked. It’s getting more and more likely that we don’t understand something fundamental about the nature of the cosmos, and the implications are exciting. Perhaps the acceleration of the universe is quicker now than it was in the early universe. Maybe dark energy isn’t constant. Maybe we need a new theory of gravity. Or maybe there’s an entirely different factor we haven’t yet considered. It’s a puzzle, and piecing it together is going to be vital to understanding how the universe works on the largest scales.
3. Quantum computing isn’t the future. It’s the present.
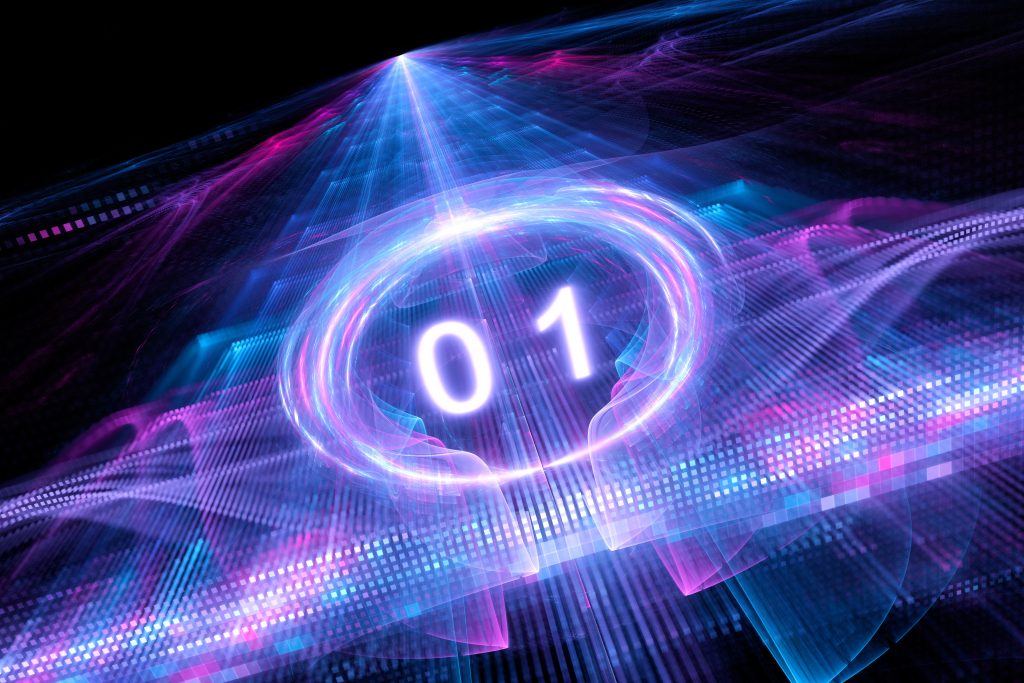
In 1994, Peter Shor, an applied mathematician working at Bell Labs, showed that in some scenarios, quantum computers could drastically outperform classical computers. Since then, there’s been a global effort to make quantum computing a reality. And it’s here.
The best quantum supercomputers of today can do some impressive stuff. They are useful, for example, to researchers studying the boundaries between quantum and classical physics. They can simulate quantum effects in condensed matter materials and help chemists study the behaviour of new compounds. Quantum computers are also improving quantum measurement (known as metrology), a tool used in precision sensors and health care devices.
The business case for quantum computing is growing too, solving optimization problems like last-mile delivery strategies for shipping companies and providing new analysis tools for the finance world.
Quantum computing has matured. What it can do today is miles ahead of quantum computers a decade ago, and there’s no telling what it will achieve in the next decade.
The key challenge in quantum computing now is to make quantum systems more stable. Quantum computing relies on entanglement (by which two particles are connected in such a way that measuring one particle tells you something about the other instantaneously). Entanglement is notoriously fragile – interference from the outside world can cause entanglement to break down via a process called decoherence. Research is ongoing to find better methods to deal with decoherence and quantum “noise,” a field of study known as quantum error correction.
With better error correction, quantum computing could revolutionize large-scale simulations and have wide-ranging implications for cryptography and cybersecurity. (In the meantime, maybe just avoid using qwerty123 as your password.)
4. “It’s teleportation, Jim, but not as we know it.”
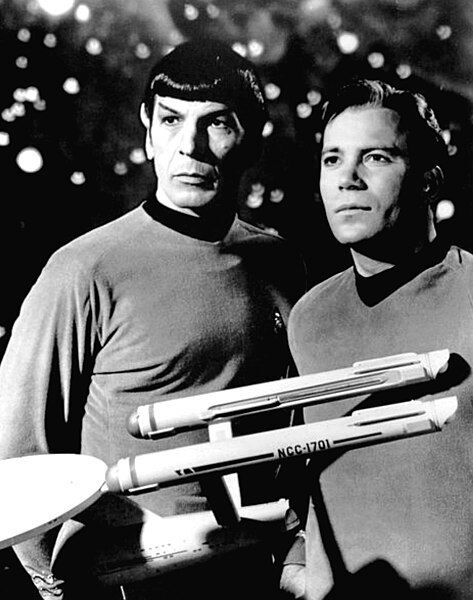
Quantum teleportation is real. Theorists first proposed the possibility in 1993. Four years later, research groups led by physicists Sandu Popescu and Anton Zeilinger achieved it in the laboratory. Since then, scientists have tested quantum teleportation at longer and longer distances.
Unlike sci-fi depictions, quantum teleportation moves information rather than physical objects. It’s like a quantum fax machine. (Do people still use those? Email. It’s quantum email.)
To be more precise, quantum teleportation is an extension of entanglement. Imagine two people, Alice and Bob. Each has one particle of an entangled pair. Alice also has a third particle, whose quantum state she wants to share with Bob. Through a series of measurements, Alice can precisely replicate the state of her third particle on Bob’s half of the entangled pair. In the process, Alice’s third particle will be transformed into a new state, leaving only Bob with the quantum information Alice used to have.
Quantum teleportation does not work faster than light. The process still requires Alice to tell Bob via normal, subluminal communication channels which quantum measurement he must perform to receive the information. The principle of quantum teleportation, however, makes it an exciting new tool with applications in quantum computing.
5. The night sky has changed (and is always changing).
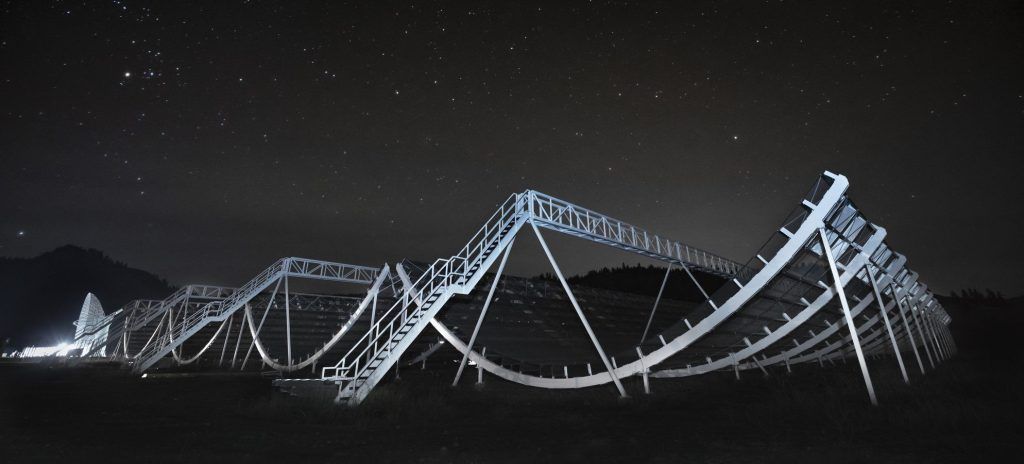
In 2007, astronomers found a mysterious new radio pulse coming from the night sky, unlike anything anyone had seen before. Then they found more. Some were one-time events. Others repeated at regular intervals. All of them were puzzling. They’re called fast radio bursts (FRBs), and in the years since, instruments like Canada’s CHIME telescope have detected hundreds of them.
Now we finally know what at least some of them are. In 2020, astronomers found an FRB within our own galaxy that corresponds to a known magnetar: a fast-spinning neutron star with a powerful magnetic field. So at least some FRBs come from magnetars. But the case isn’t closed yet, because some FRBs just don’t fit the magnetar hypothesis. Astronomers are still working to explain these outliers.
The hunt for FRBs is part of the growing field of transient astronomy: studying things that change in the night sky over short time spans (astronomically speaking). You can expect much more news from transient astronomy in the coming years. CHIME is being expanded and improved, and other new radio observatories, like the Square Kilometer Array, are in the works. Transient optical astronomy is about to get a big boost too, with the brand new Vera Rubin Observatory coming online soon. It will scan most of the night sky every few days, looking to catch anything that moves or changes. It’s going to be a wild ride.
6. We’re making (super)cool new materials.
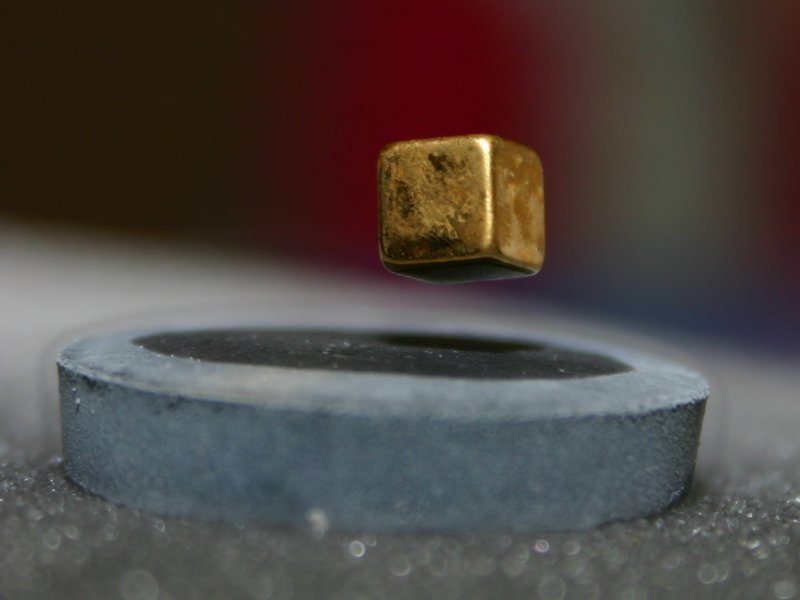
Peter nussbaumer, CC BY-SA 3.0, via Wikimedia Commons[/caption]
Materials science has advanced dramatically in recent decades, due to contributions from condensed matter physics. In 1995, the discovery of the first Bose-Einstein condensate blew the doors open. This supercooled material makes quantum effects apparent at macroscopic levels: the entire condensate acts like a giant single atom. It’s very cool.
In 2004, researchers isolated another exciting new material. Called graphene, this immensely strong, light material is only one layer of atoms thick, making it an excellent material for semiconductors and electronics.
Condensed matter physics has also revealed more about the science of phase transitions, like ice melting to water or magnetism emerging in iron. “Emergence” refers to characteristics that manifest across an entire material that aren’t explained by the features of individual atoms or molecules, like magnetism, colour, elasticity, heat, and electric conductivity.
One particularly exciting emergent behaviour is superconductivity – the property of conducting electricity with zero resistance. It emerges only at extremely cold temperatures, though recent research has made superconductivity possible at higher and higher temperatures. Currently, the best high-temperature superconductors reliably function at about –135°C.
Other new discoveries in condensed matter include spin liquids, which exhibit long-range quantum effects, and time crystals, whose oscillating ground states create motion without energy. Today, condensed matter is the largest subfield of physics, and it’s easy to see why. It bridges the theoretical with the practical and underpins many emerging technologies.
7. Neutrinos have mass (and we can use them like telescopes).
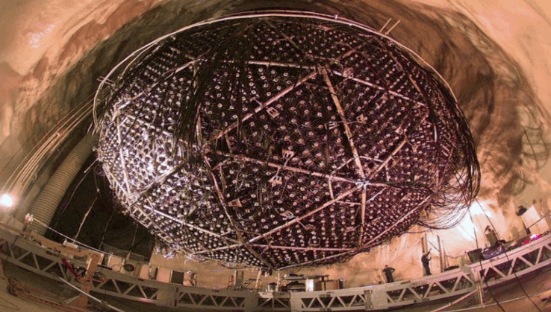
Neutrinos are among the most intriguing particles in the Standard Model. They come in three “flavours,” and they hardly interact with anything. Trillions of neutrinos pass through you every second, almost at the speed of light.
That “almost” is important. If they moved at the speed of light, they would be massless like photons. But around the turn of the 21st century, researchers in Japan and Canada discovered that neutrinos can change from one flavour to another, something only possible if they have mass (and if each flavour has a different mass).
Physicist still aren’t entirely sure why neutrinos have mass. It doesn’t seem to be due to interactions with a particle called the Higgs boson, which is where other particles get their mass. That’s because as far as we know, neutrinos only come in left-handed form (they spin in only one direction). They would need a right-handed counterpart to interact with the Higgs. The jury is still out on whether right-handed neutrinos exist – there’s no sign of them anywhere.
In the meantime, neutrinos are becoming useful. They offer a unique window on the universe, helping launch a whole new field of study known as multimessenger astronomy. We no longer have to rely only on the electromagnetic spectrum (visible light, radio waves, X-rays, etc.) to study the night sky. Instead, other “messengers,” like neutrinos and cosmic rays (protons and neutrons), reveal things photons simply can’t. Just this past summer, for example, astronomers used the IceCube neutrino detector in Antarctica to map neutrino sources in the Milky Way, presenting a picture of our galaxy as we’ve never seen it before.
8. We’ve confirmed some big theoretical ideas.
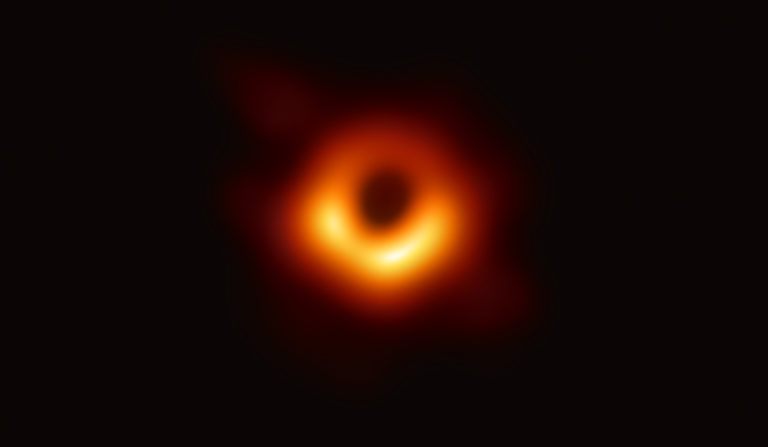
Sometimes, physicists are right! Three of the biggest physics news stories in recent memory were dramatic confirmations of existing theories. So let’s close out this list with three ways the textbooks don’t need to be rewritten.
First, CERN’s Large Hadron Collider found the Higgs boson. This particle is the pièce de résistance of the Standard Model of particle physics. It is the mechanism that gives other particles mass (except for those pesky neutrinos). Physicists predicted the Higgs boson’s existence in the 1960s, though proof only came in 2012, when the Large Hadron Collider detected the particle for the first time.
Second, LIGO (the Laser Interferometer Gravitational-Wave Observatory) directly detected gravitational waves for the first time in 2015. Einstein predicted these ripples in spacetime more than a century ago in his theory of general relativity. Like neutrinos, gravitational waves are another new messenger in multimessenger astronomy, offering an untapped resource with which to study the universe. Using LIGO, we can observe the collisions of black holes and massive stars via their gravitational effects, not just their radiation. Alternate techniques for gravitational wave detection have also made incredible progress, like the NANOGrav pulsar timing array, which in 2023 revealed a cosmic “hum” of gravitational waves in low frequencies beyond what LIGO is designed to detect.
Third, in 2019 the Event Horizon Telescope (EHT) collaboration imaged a black hole for the first time. Using a method called very-long-baseline interferometry, the EHT linked together telescopes from around the world to create a virtual radio telescope the size of the planet. This allowed them to capture images of the swirling accretion disk surrounding a supermassive black hole at the centre of Galaxy M87, as well as images of our own galaxy’s central black hole. The EHT’s techniques have only improved since then, so we can definitely expect more detailed black hole observations in the years to come.
A lot has changed since your school days, eh? This list is just a taste of the ongoing revisions and revolutions in physics. There’s plenty more making waves, from holography to string theory, causal inference to quantum chromodynamics, and quantum field theories to dark matter. Everything’s always changing!
Want to keep up with the latest news? Sign up for our newsletter here. If you do, we promise, at the very least, you’ll never run out of interesting things to say at parties!
About PI
Perimeter Institute is the world’s largest research hub devoted to theoretical physics. The independent Institute was founded in 1999 to foster breakthroughs in the fundamental understanding of our universe, from the smallest particles to the entire cosmos. Research at Perimeter is motivated by the understanding that fundamental science advances human knowledge and catalyzes innovation, and that today’s theoretical physics is tomorrow’s technology. Located in the Region of Waterloo, the not-for-profit Institute is a unique public-private endeavour, including the Governments of Ontario and Canada, that enables cutting-edge research, trains the next generation of scientific pioneers, and shares the power of physics through award-winning educational outreach and public engagement.